Basic Studies in Support of the International Linear Collider (ILC) Project
Cornell University’s superconducting cavity program is one contributor to the global collaborative effort on critical SRF R&D for the ILC. Our goal is to produce high-Q Eacc > 35 MV/m superconducting niobium cavities set forth by the ILC Global Design Effort. This work continues today supporting the ILC S0 goal of reproducibly producing high-Q Eacc > 35 MV/m superconducting niobium cavities set forth by the ILC-GDE [1].NEW QUENCH DETECTION METHOD
We are following the general ILC R&D strategy for raising the high-gradient cavity yield by locating the quench producing defects in low low-performing cavities, followed by optical inspection and repair. In the past year we have assembled and demonstrated a new and efficient quench-spot location system using 2nd sound in superfluid helium. This system employs an array of oscillating superleak transducers to detect the second sound waves generated during quench. The distance between three or more transducers and the quench-spot is determined by measuring the time-of-flight between the arrival of the second sound wave at each transducer and the cavity quench location. With this information the quench location can be unambiguously determined in three dimensions.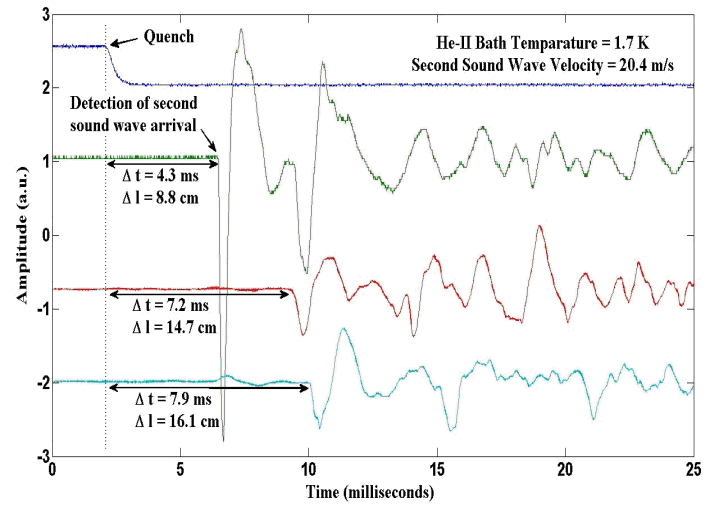
Figure 1: A quench event. Four traces are shown: 1) the upper trace is the quenching-cavity transmitted power, 2-4) the lower traces are transducer second sound signals.
CAVITY REPAIR
Cornell’s work with single cell cavities [2] shows that “bump-like” defects can be fixed with additional etching, e.g. BCP. However, there is mounting evidence that there are pit-like defects which cannot be fixed with heavy EP. Several ideas are currently under investigation to fix such defects: centrifugal barrel polishing, local grinding/polishing, electron-beam melting, laser melting, tumbling, etc. We employed a simple cavity tumbling apparatus which is cheap and simple to operate. The apparatus was initially developed to tumble two-cell cavities for the ERL Injector cryomodule [3]. It was modified to tumble cavities with one to nine cells. In this configuration the Cornell tumbling apparatus removes ~10 µm of surface material per day at the equator weld. This removal rate is significantly less than centrifugal barrel polishing but the system is lower in cost and easier to implement.ALTERNATE CAVITY DESIGNS
Cornell is pursuing alternate cavity shapes which are designed to minimize the ratio of surface magnetic field to accelerating gradient in order to increase the maximum accelerating gradient limited by the RF critical field of niobium. One proposed shape, the Cornell reentrant design [4] achieved accelerating gradients exceeding 50 MV/m in single cell tests at Cornell [5] after post-purification (at Cornell), tumbling (at KEK) and EP (at KEK). Implementing this achievement in 9-cell cavities is the area of active work. A joint venture between Advanced Energy Systems, Inc., and Cornell has fabricated two multi-cell reentrant cavities: a 9-cell and a 3-cell. The 9-cell reentrant cavity’s accelerating gradient increased dramatically after we employed second sound detectors to locate the gradient limiting pit-defect, tumbling to repair the defect, and vertical electropolishing. The lower Q is most likely due to excess hydrogen contamination accumulated during tumbling. We found similar low-Q behavior with the 3-cell reentrant cavity which was also tumbled to smooth out the equator weld region and hydrogen-degassed with the standard temperature and time. The Q-disease showed up in the 3-cell after parking the cavity in the dangerous temperature zone (100 – 150 K).ALTERNATE PROCESSING
Electropolishing (EP) is a critical treatment step for the production of niobium cavities with accelerating gradients > 35 MV/m. The majority of EP facilities are using the continuous EP method developed at KEK [6] with the cavity held in the horizontal orientation. We have developed an alternative method, vertical electropolish, which was designed to be less expensive and easy to install to support the mass-processing of cavities during ILC construction, refer to [7, 8]. The continuous VEP procedure:- Eliminates rotary acid seals
- Eliminates sliding electrical contacts
- Eliminates the cavity vertical/horizontal position control fixturing
- Simplifies the acid pluming/containment
NEW VENDOR CAVITY EVALUATION
To build the ~14,000 cavities required for the ILC each of the three world regions must have a sizable industrial base of qualified companies to draw cavities from [1]. We have been investigating new cavity vendors for this production.REFERENCES
- www.linearcollider.org
- Z.A. Conway, et al, “Defect Location in Superconducting Niobium Cavities Cooled with He-II Using Oscillating Superleak Transducers,” PAC 2009, Vancouver, British Columbia, Canada, May 2009, TU5PFP044
- R.L. Geng, et al, “Fabrication and Performance of Superconducting RF Cavities for the Cornell ERL Injector,” PAC 2007, Albuquerque, New Mexico, USA, WEPMS007, Pg. 2340 (2007); www.jacow.org
- V. Shemelin, et al, “Optimal Cells for TESLA Accelerating Structure,” Nucl. Instr. and Meth. in Phys. Res. A 496 Pg. 1-7 (2003)
- R.L. Geng, et al, “High Gradient Studies for ILC wit Single-Cell Re-Entrant Shape and Elliptical Shape Cavities Made of Fine-Grain and Large-Grain Niobium,” PAC 2007, Albuquerque, NM, USA, June 2007, WEPMS006, Pg. 2337; http://www.jacow.org
- K. Saito, et al, “Electropolishing of L-Band Cavities,” in The 4th International Workshop on RF Superconductivity, Tsukuba, Japan, Pg. 635-695 (1989)
- R.L. Geng, et al, “Vertical Electropolishing Niobium Cavities,” The proceedings of the 12th International Workshop on RF Superconductivity, Ithaca, New York, July 2005, THP04
- A.C. Crawford and H.S. Padamsee, “A Procedure for Vertically Oriented Multicell Niobium Radiofrequency Cavities,” PAC 2009, Vancouver, British Columbia, Canada, May 2009, TU5PFP050