SRF News: 2010
Fabrication of seven-cell cavities for the international ERL cryomodule collaboration (07/10)
Cornell is a critical contributor to the international ERL cryomodule collaboration (Daresbury/Cornell/DESY/Rossendorf/LBNL/TRIUMF) which develops an optimized cavity/cryomodule solution for ERL facilities. This week, Cornell’s SRF group completed fabrication and testing of both seven-cell superconducting RF cavities for this cryomodule. This is an important milestone for this collaboration as well as for Cornell’s ERL which will have similar 7-cell cavities.The cavity sections from the first to the last equator were cut from two seven-cell superstructure cavities provided by DESY. The outer half-cells and associated beam pipes (end groups) are of a new design developed by LBNL, Daresbury and Cornell. Their geometries were optimized to facilitate the propagation of higher order mode power to ferrite-lined beam-pipe loads, identical to those used in the Cornell ERL injector cryomodule. One of the two end groups is fitted with an input power coupler port that will accommodate a slightly modified version of the Cornell ERL injector coupler.
Upon completion of the mechanical design, the end cells were fabricated and electron-beam welded to the center sections. Subsequently, the cavities have been tuned to achieve desired resonant frequency and field flatness of the π-mode. As the cavities will operate in CW mode with moderate gradients, only BCP and HPR treatments were used for the cavity preparation. After series of vertical tests, both cavities achieved accelerating gradients in excess of 18 MV/m. Following the successful vertical testing, titanium helium jackets were welded to the cavities; the structures went through the final cleaning cycle and are ready to be shipped to Daresbury to be assembled in the cryomodule.
Helium liquefaction at Cornell's SRF group (03/20)
On Friday, March 20, 2010, Cornell's SRF group for the first time liquefied helium that had been recaptured from a cryogenic cavity test. The cost of helium has strongly increased over the last few years, and the cost reduction associated with not venting used helium to the atmosphere is a large step forward for Cornell's SRF group. Saving this limited, and not replenishing resource also contributes to the sustainability of science.Cornell's SRF group has three, 15 feet deep, magnetically shielded, and radiation-protected cryogenic test pits for the cold test of superconducting cavities. In these pits, cavities are cooled down to as low as 1.6K to analyze the electromagnetic fields that can be excited in the superconducting state. Cooling a 1m long cavity and performing the required analysis needs about 3000 liters of liquid helium. The plant is capable of liquefying 30 liquid liters per hour, requiring 4 days to prepare helium for a test, well within a test's setup time.
Helium is so light that it is not retained by the earth's gravitational potential and escapes into space. Because helium, as a nobel gas, is not bound in chemical compounds, the only helium left on earth stems from nuclear decay within the earth. Helium is therefore a non-replenishing, limited resource, which factors into the rise in cost; and capturing used helium therefore contributes to the sustainability of scientific research.
Temperature dependence of the superheating field in niobium measured (03/12)
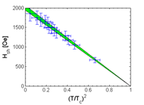
Figure: Plot of the superheating field data versus (T/Tc)2, where the sample's critical temperature was Tc=8.83 K. The green cone shows the Ginzburg-Landau prediction. The cone's width results from measurement uncertainty in a model parameter. The measured data agrees well with the model to within measurement errors..
Improved ERL injector cryomodule completed by the SRF group (02/16)
Cornell's prototype ERL injector cryomodule had to be completely dismantled for the following improvements:1. the Q0 of the superconducting two cell cavities was only approximately 4*109, instead of the anticipated 2*1010. Cavities were therefore cleaned with high-pressure water, and some by buffered chemical polishing.
2. the 6 higher-order mode absorbers had been shown to charge up during electron-beam operation, leading to unintended deflections of the beam. These absorbers therefore had to be simplified, so they do not expose chargeable surfaces to the beam. Furthermore, their design was changed so strong tensions are avoided under cool down. Improving this cryomodule has been a large effort at the SRF laboratory and has taken approximately 4.5 months. However, on Monday February 8, 2010, the complete accelerating module has been moved from the SRF group to the accelerator test area in Wilson laboratory where it will accelerate electron beam about one month later.